Aptamers as the new antibodies: A new approach to detecting and neutralizing pathogens
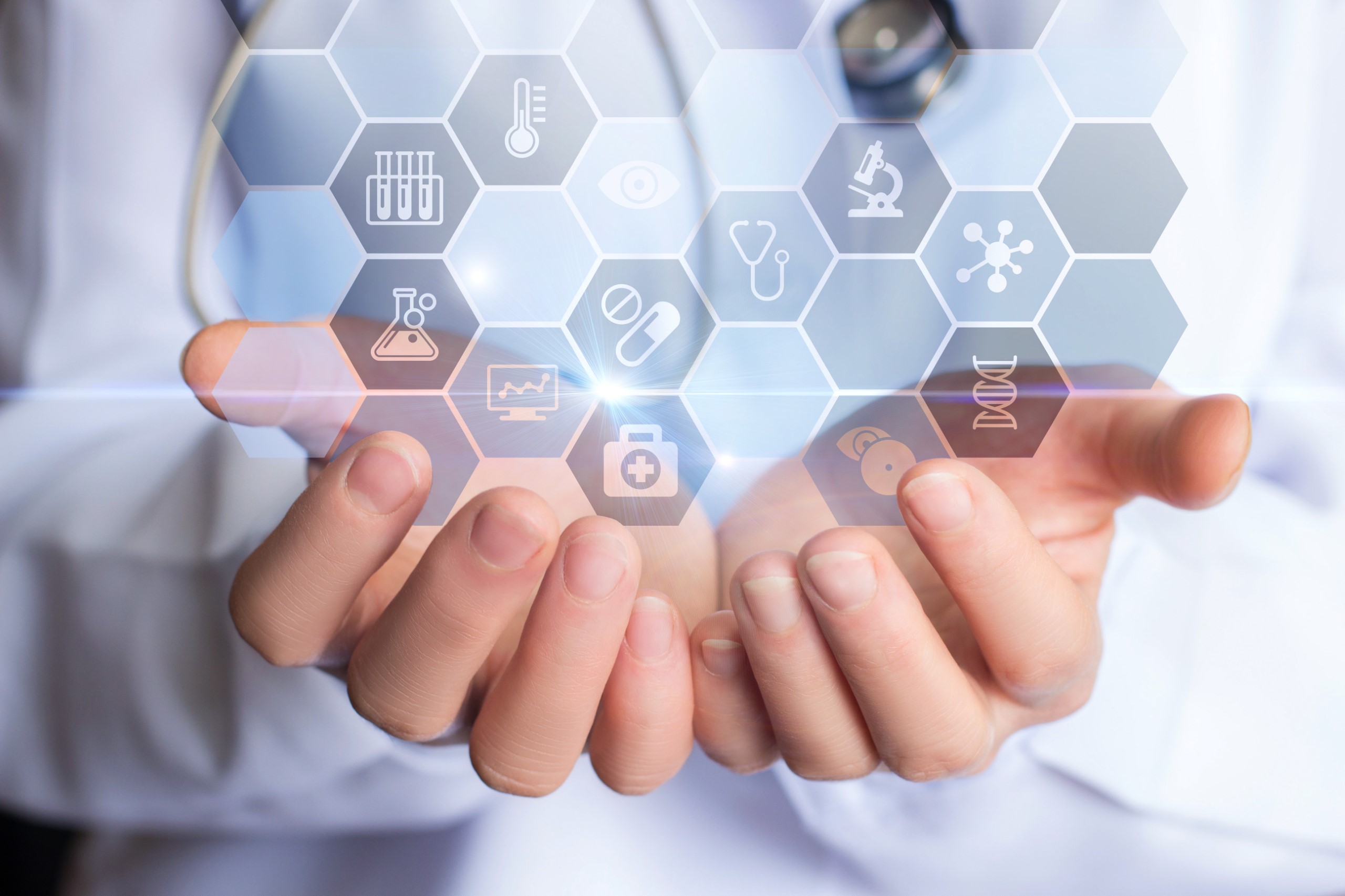
Antibodies – ubiquitous in biology research, powerhouses for at-home diagnostics, and recently famous as COVID-19 therapeutics, these proteins are considered critical jacks-of-many-trades for the biotech industry. But antibody production is slow, costly, and has storage limitations, and the strong target-binding behavior of antibodies precludes their use in some applications.
Then there are aptamers, the newer kids on the block. These short, single-stranded RNA or DNA molecules may be thought of as nucleotide analogs of antibodies. Aptamers can bind to many types of targets, ranging from simple inorganic molecules to large protein complexes, and even entire cells. They have a wide variety of applications, including as affinity reagents, bioimaging probes, and in sensing, environmental surveillance, clinical diagnostics, and therapeutics.[1] Aptamer synthesis is faster, easier, and cheaper than antibody production, and given their demonstrated specificity and improving target affinity, aptamers have the potential to be an alternative to antibodies for some diagnostic and therapeutic purposes.
Every moment counts during a public health emergency, and technologies that support faster and less costly production of medical countermeasures—like diagnostics and therapeutics—are needed to prepare for and quickly respond to health security threats. With this in mind, it is important to consider how aptamer-based technologies can address some of the shortfalls of antibody-based solutions, and where more research is needed to better understand their utility.
Production
Antibody production involves animals and cell culture. In general, animals like mice or rabbits are immunized with the target of interest, then, for monoclonal antibodies, antibody-producing cells are isolated and immortalized in cell culture for continuous antibody production. This is a time-and labor-intensive process, and scaleup requires maintenance of large quantities of cell culture.
In contrast, aptamer production does not require biological systems, making manufacturing scaleup more straightforward. Additionally, recent advances have made selection more rapid and scalable. Aptamer synthesis occurs by chemical reactions in vitro—not by using animals or cell culture. After synthesis, the resulting diverse set of aptamers goes through a selection process, which also happens in vitro, to find the best performing candidate for a particular application. This general process is called SELEX (systematic evolution of ligands by exponential enrichment),[2] and recent work has demonstrated that aptamer selection can occur in the environment of the desired application and can happen at a rapid throughput of tens of millions of candidates per week with minimal labor.[3]
Although new protein synthesis platforms have demonstrated the potential for vastly enhanced protein (and, specifically, antibody) generation at large scale,[4] the potential cost savings of aptamer production makes further development of aptamers as sensing and therapeutics reagents compelling. In addition, the proliferation of nucleic acid synthesis technologies — that enable both large-scale manufacturing and the generation of ever-longer high quality and high purity nucleic acids — makes aptamers very attractive from a regulatory and business perspective.
Diagnostics
Because the natural function of antibodies and aptamers is to bind their targets, both have great utility in diagnostic platforms to detect, and sometimes quantify, biomarkers of interest. For example, antibody-based platforms are very common in the at-home diagnostics space, with COVID-19 antigen tests and home pregnancy tests as two well-known examples. Antibodies are great for this purpose because, in general, they strongly bind their target and do not easily let go (that is, they have high avidity).
Aptamers suffer from a reputation of having lower avidity, but what seems like a downside actually can be an advantage. As aptamers bind and release their targets, they can act as switches in continuous monitoring applications. Such applications include continuous glucose, cortisol, or lipid monitoring; control of dosing for chemotherapeutics and analgesics; and therapeutic applications where drug activity needs to be tightly managed. Indeed, this is where the greatest excitement in work with aptamers exists and where many researchers are focusing efforts.[5]
Research is also underway to develop aptamers with better avidity, with the ability to bind small molecules through incorporation of non-natural nucleic acid bases, and new methods of aptamer selection.[6] These advances could broaden and enhance the utility of aptamers in diagnostic applications. Additional exciting work is being done with multi-valent aptamer complexes that can detect “big” targets, like large molecules, whole viruses, and even whole bacteria. In this application, multiple aptamers are generated to bind various parts of the target, or multiple targets, and work synergistically for detection.[7][8]
Therapeutics
Monoclonal antibody therapies have made a big impact in the fight against COVID-19 by providing pre-exposure protection for people who are immunocompromised or for whom the COVID-19 vaccine is not recommended and as a treatment for COVID-positive patients with mild or moderate symptoms. But those treatments were far from the first ones available. In fact, the U.S. Food and Drug Administration (FDA) approved the 100th monoclonal antibody therapy in April 2021—with 50 of those approvals happening only in the prior six years[9] — emphasizing that these therapies are clearly staking their claim on the market.
Similarly, Aptamers could be used as a pathogen neutralizing therapy, but, as a newer technology, aptamers understandably lag antibodies in development and market presence. There is currently only one approved aptamer therapy (Macugen), indicated for treatment of age-related macular degeneration. One challenge holding aptamers back from therapeutic success is their short half-life in the body. Because aptamers are small and are made up of nucleic acids, they are highly susceptible to metabolic digestion and are quickly cleared from the body. Overcoming these hurdles, or figuring out how to benefit from them, will be key to success in the therapeutic space.
Despite their current minimal market presence, aptamers may be promising candidates for therapeutic development. Aptamers can activate the function of target receptors, and multivalent aptamer complexes (noted previously for the detection of large targets) are being explored as drug delivery mechanisms for specific cells or tissues, replacing lipid rafts and other such molecular targeting strategies.[10] With relevance for medical countermeasure development, a recent review by M. Stephens (2022) provides in-depth coverage of research into the use of aptamers as therapeutics for inflammation and infection.[11]
Aptamer technology, despite the many hurdles to overcome, may be poised to bring innovation and new capabilities to the development of new medical countermeasures. Continued investment in this technology will be critical to its successful commercialization.
[1] Kaur H, Bruno JG, Kumar A, Sharma TK. Aptamers in the therapeutics and diagnostics pipelines. Theranostics. 2018;8(15):4016-4032. doi:10.7150/thno.25958
[2] For an overview of different SELEX variations, see, for example: Zhang Y, Lai BS, Juhas M. Recent advances in aptamer discovery and applications. Molecules. 2019;24(5):941. doi: 10.3390/molecules24050941
[3] See, for example, work from Stanford (Soh Lab), University of California Santa Barbara (Plaxco Lab) and University of California San Diego (Wang Lab)
[4] Platforms developed by Lumen Biosciences, Dyadic, Twist Biosciences, among others are good examples of new protein synthesis technology
[5] Among many examples are work from the University of California Berkeley (Javi lab), University of California San Diego (J. Wang lab), Northwestern University (Rogers lab), University of California Santa Barbara (Plaxco lab), Stanford (Soh lab), and Rutgers University (Javanmard lab)
[6] See, for example, work from the University of California Santa Barbara (Plaxco lab) and Stanford (Soh lab), among others
[7] Wang Z, Yang X, Lee NZ, Cao X. Multivalent aptamer approach: Designs, strategies, and applications. Micromachines. 2022;13(3):436. doi:10.3390/mi13030436
[8] Kwon PS, Ren S, Kwon SJ, et al. Designer DNA architecture offers precise and multivalent spatial pattern-recognition for viral sensing and inhibition. Nature Chemistry. 2020;12(1):26-35. doi:10.1038/s41557-019-0369-8
[9] Mullard A. FDA approves 100th monoclonal antibody product. Nature Reviews Drug Discovery. May 5, 2021. https://www.nature.com/articles/d41573-021-00079-7
[10] As one example: Yoo H, Jung H, Kim SA, Mok H. Multivalent comb-type aptamer-siRNA conjugates for efficient and selective intracellular delivery. Chemical Communications. 2014;50(51):6765-6767. doi:10.1039/c4cc01620c
[11] Stephens M. The emerging potential of aptamers as therapeutic agents in infection and inflammation. Pharmacology & Therapeutics. 2022; 108173. doi.org/10.1016/j.pharmthera.2022.108173